Stanford researchers discover how brain regions work together, or alone
Posted By News On February 2, 2014 - 6:30pm

Stanford researchers may have solved a riddle about the inner workings of the brain, which consists of billions of neurons, organized into many different regions, with each region primarily responsible for different tasks.
The various regions of the brain often work independently, relying on the neurons inside that region to do their work. At other times, however, two regions must cooperate to accomplish the task at hand.
The riddle is this: what mechanism allows two brain regions to communicate when they need to cooperate yet avoid interfering with one another when they must work alone?
In a paper published today in Nature Neuroscience, a team led by Stanford electrical engineering professor Krishna Shenoy reveals a previously unknown process that helps two brain regions cooperate when joint action is required to perform a task.
"This is among the first mechanisms reported in the literature for letting brain areas process information continuously but only communicate what they need to," said Matthew T. Kaufman, who was a postdoctoral scholar in the Shenoy lab when he co-authored the paper.
Kaufman initially designed his experiments to study how preparation helps the brain make fast and accurate movements – something that is central to the Shenoy lab's efforts to build prosthetic devices controlled by the brain.
But the Stanford researchers used a new approach to examine their data that yielded some findings that were broader than arm movements.
The Shenoy lab has been a pioneer in analyzing how large numbers of neurons function as a unit. As they applied these new techniques to study arm movements, the researchers discovered a way that different regions of the brain keep results localized or broadcast signals to recruit other regions as needed.
"Our neurons are always firing, and they're always connected," explained Kaufman, who is now pursuing brain research at Cold Spring Harbor Laboratory in New York. "So it's important to control what signals are communicated from one area to the next."
Experimental design
The scientists derived their findings by studying monkeys that had been trained to make precise arm movements. The monkeys were taught to pause briefly before making the reach, thus letting their brain prepare for a moment before moving.
Remember, the goal was to help build brain-controlled prostheses. Because the neurons in the brain always send out signals, engineers must be able to differentiate the command to act from the signals that accompany preparation.

Matthew T. Kaufman and a neuroscience researcher.
(Photo Credit: Matthew T. Kaufman)
(Photo Credit: Matthew T. Kaufman)
The muscle readings enabled the scientists to ascertain what sorts of signals the arm receives during the preparatory state compared with the action step.
The brain readings were more complex.
Two regions control arm movements. They are located near the top center of the brain, an inch to the side.
Each of the two regions is made up of more than 20 million neurons. The scientists wanted to understand the behavior of both regions, but they couldn't probe millions of neurons. So they took readings from carefully chosen samples of about 100 to 200 individual neurons in each of the two regions.
During experiments the scientists examined the monkeys' brain readings at two levels.
On one level, they considered the activity of individual neurons – how quickly or slowly the neurons fired signals.
At a higher level, the scientists also identified patterns of changes in the activity of many neurons. This is a relatively new technique for neuroscience, called a population and dimensionality analysis. Its goal is to understand how neurons work together in entire regions of the brain.
Hunting for the signal
The key findings emerged from understanding how individual neurons worked together as a population to drive the muscles.
As the monkey prepared for movement but held its arm still, many neurons in both of the motion-control regions registered big changes in activity.
But this preparatory activity did not drive the movement. Why?
The scientists realized that, during the preparatory stage, the brain carefully balanced the activity changes of all those individual neurons inside each region. While some neurons fired faster, others slowed down so that the entire population broadcast a constant message to the muscles.
But at the moment of action, the population readings changed in a measurable and consistent way.
By looking at the data, the scientists could correlate these changes at the population level to the flexing of the muscles. This change at the population level differentiated action from preparation.
Broader ramifications
The Stanford researchers put great effort into the mathematical analysis of their data. They had to be sure that each of the two populations of neurons exhibited the key muscle-controlling changes in activity when (and only when) the muscles flexed. This was the signal they had set out to find.
Kaufman said he was about one year into what turned out to be a three-year project when he realized there might be broader ramifications to this population-level and dimensionality identification idea.
He was presenting an early version of the brain-to-muscle results at a scientific conference when a question from one his peers caused him to think. He had found population-level signals between the brain regions and the muscles. Did the two brain regions, each partially in control of the action, couple and uncouple with each other in a similar way?
"I started the analysis in my hotel room that night at one a.m.," Kaufman recalled. "Soon enough, the results were clear."
"The serendipitous interplay between basic science and engineering never ceases to amaze me," said Professor Shenoy, who is also professor of neurobiology (by courtesy) and bioengineering (affiliate), and a Bio-X faculty member. "Some of the best ideas for the design of prosthetic systems to help people with paralysis come from basic neuroscience research, as is the case here, and some of the deepest scientific insights come from engineering measurement and medical systems."
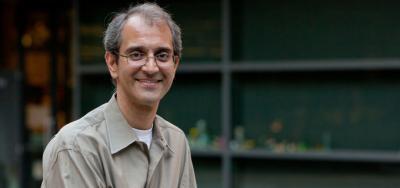
Krishna Shenoy is a Stanford electrical engineer working on brain caroled prosthetic devices.
(Photo Credit: Joel Simon)
Source: Stanford School of Engineering
No comments:
Post a Comment
Please leave a comment-- or suggestions, particularly of topics and places you'd like to see covered